Introduction
The information below has been prepared by Robert Gourlay, RFD, B.Appl.Sc, M.Appl.Sc., based on over 30 years of research into deep, fractured rock groundwater sources, and over 25 years of groundwater bore location mapping and exploration to depths beyond 300m.
Nearly 100% of the water used, at a global scale, has its origin in the water supplied by the hydrological cycle. That is, water that is collected in dams and drawn from shallow bores. The reliance on surface water flows and the shallow groundwater system is a significant issue for the sustainable management and future development of water supply to residents and agricultural industries.
Groundwater is important for food security, human health and the health of soil and water ecosystems (eg. rivers and lakes). The over-exploitation of the surface water flow in rivers and the shallow groundwater systems is now threatening water supply security and therefore food security, at regional, national and global scales.
This over exploitation is primarily due to the myths about how water was formed on the Earth and the availability of groundwater resources. Below is a diagram that explains the layered structure of groundwater, below the Earth’s surface.
Maps of the groundwater bore system within Australia show that most of the groundwater extraction (possibly 99%) is drawn from the shallow groundwater aquifers that represents about 2% of the available groundwater sources within an area. This extraction concentration is unsustainable. This paper proposes utilising the deep fractured rock waters that are confined and separated from the surficial groundwater systems that water users depend on for water supply. This deep, fractured rock water is new water and will sustain regional water users during times of drought and allow for population and industry expansion.
Earth generated water
The classic and traditional models used by hydrologists only explains the shallow groundwater system flows and does not include a deep, confined groundwater system. Below are examples of the traditional Hydrological Cycle Model and the Earth Generated Water Model.
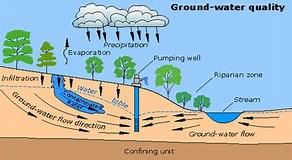

Economic, social and ecological issues of groundwater systems
Ecological and socioeconomic aspects of shallow groundwater systems have changed since the 1950’s due to increased extraction rates, primarily to support agricultural irrigation. The major environmental pressures (mainly anthropogenic or human ones) impact the quantity and quality of groundwater resources and the state of subsurface ecosystems. It is expected that the environmental pressures on groundwater will continue, at least until 2025 (forecasted wet year), unless new environmental policies for the use of shallow groundwater systems change under Local Government Development Application controls.
The world population increase, and the general rise of water demand constitutes one of the major environmental pressures on shallow groundwater ecosystems. Specific human activities leading to the depletion of groundwater reserves include agricultural practices, intensive agriculture enterprises (eg. chicken farms, feedlots and abattoirs), road construction and maintenance, urbanisation expansion and the demand for increased domestic and public drinking water. Also, various industrial activities such as mining, and the rise in tourism infrastructure increased pressure on new water supplies. However, the increasing drying of surface soils and shallow groundwater flows since the 1950’s is contributing to the surface and shallow groundwater water supply crisis.
The overload of shallow groundwater aquifers with pollutants derived from agriculture (fertilisers, insecticides and pesticides), industry (ie. release of hydrocarbon chemicals, especially spills), waste and industrial waters, domestic and industrial landfills, infiltration of pollutants from surface and the intrusion of saline water, affect shallow groundwater quality. The dangerous increase in contaminated subsurface sites with chemicals and microbial pathogens brings health risks to humans and domestic or housed animals. Changes of chemical conditions (ie. oxidation) in groundwater zones, changes of biological diversity, vegetation changes with modification of agriculture practices and impacts at the biosphere scale, such as the increase in the concentration of nitrous oxides in the atmosphere, all impact groundwater ecosystems.
Groundwater ecosystems must be better investigated and understood in the region. Economic, social and ecological knowledge has to be combined in order to achieve meaningful policies for the sustainable development of groundwater reserves and for the protection of shallow, subsurface groundwater ecosystems. Practical measures and ideas for the development of Local Government policies up to the 2025 time-horizon should improve the sustainable usage of the regions groundwater resources.
Deep, confined, fractured rock groundwater supplies
The Earth is a dynamic process of change however it is the presence of water that defines the nature of life on Earth and the ecological process in nature. Groundwater systems have been forming in the Earth for more than 4 billion years from a water forming process below the Earth’s crust. This process is best described in a book (A Voyage of Discovery) by Prof. Lance Endersbee AO (former Vice Chancellor of Monash University, Victoria Australia) This Earth generating water process is perpetual, and it is this process that created the oceans, the hydrological cycle, the rivers and the layers of buried groundwater systems in ancient, buried sediments and rock fractures. These buried groundwater systems in the crust are also dynamic due the Earth’s continuous expansion process and associated volcanic and earthquake activity.
Groundwater systems vary in size, structural complexity and age depending on tectonic and geological processes over millions of years. There are small-scale systems (from metres to hundreds of metres) to large complex systems, sometimes spanning kilometres of galleries and fissures below the Earth. Also, large aquifers at the scale of kilometres, encompassing alluvial plains or a whole river sector within a watershed (catchment), form the shallow, regional or basin groundwater systems, that are particularly important for water management.
The subsurface hydrosphere can be characterised globally as a giant groundwater arena where the layers of the Crust are very dynamic, forming groundwater sources (ie. new water from below the Crust) to groundwater sink systems where water is consolidated in confined spaces. Water from these spaces can be upwardly flowing to the shallow groundwater systems, including outlets in the ocean (plumes), mineral springs, geysers, etc. The water storage residence time in shallow groundwater systems or superficial alluvial aquifers can vary from several days to a few years depending on the amount and frequency of rainfall events, and upward flows from the deep groundwater systems.
That is, the deep groundwater systems or the subterranean domain behave like a leaky source system for the shallow groundwater systems and the ocean. This means that the renewal of the water, proceeds at slow rates and the storage residence time in the deep groundwater sources can reach thousands of years. Finally, within the very deep Earth layers (2–5 km deep), the fluid dynamics are slow, so we can use the term inactive groundwater where energy and matter remain stored for millions of years. The total volume of the deep groundwater within the Earth’s crust is thought to be more than ten to fifty times that of the active or dynamic (upper) groundwater sources. However, all of these systems (active and inactive) are interconnected in time and space and dynamic in nature. For example, the shallow groundwater systems can change within months or years depending on climatic conditions and rates of groundwater extraction for food production and other industries. However, it is the renewal of water within the Earth that accounts for the continuous rise in ocean levels.
In this context, the extraction of deep groundwater for both urban residents and food producers, I am mainly referring to the active groundwater systems within 500m from the Earth’s surface. Specifically, I focus on the groundwater zone between 300-500m where sustained yields can vary from 50,000- 250,000 litres per hour (1.2 – 6 megalitres per day). This system is continuously recharged from the deeper layers of this groundwater system, and these upward pressure flows can sustain the shallow groundwater systems in a dynamic equilibrium.
Groundwater quality
The mineral and chemical composition of groundwater affects its suitability for different uses and this quality varies with depth from the surface. For example, water quality in the shallow groundwater systems to 200m depth can be affected by salinity, hardness (accumulation of carbonates) and chemicals (contaminants) used in agriculture and other land or industrial uses (eg. mineral and gas extractions).
In the shallow groundwater aquifers (such as the alluvial sediments down to about 60m along the Peel River irrigation zone) micro-organisms in these soil and water systems are responsible for the major turnover of energy and matter. They play a key role in weathering and formation of minerals and they store in their biomass important quantities of carbon, nitrogen and phosphorus. Moreover, they contribute to the development in the subsurface of microhabitats chemically distinguished by their redox reactions. In the context of strong human impacts on the environment, the high purification potential of groundwater ecosystems is of increasing interest and importance. Micro-organisms provide this purification service generally, however when they are impacted by compaction of soils and the input of chemical fertilisers, insecticides and herbicides the water quality of the groundwater flows in these shallow aquifers can be adversely affected.
However, the groundwater in the zone from 300-500m is generally of a spring water (potable) quality, with excellent mineral and chemical (pH/ORP) values. Much of this deep, confined groundwater has never reached the Earth’s surface and the age of the water may be millions of years old. However, significant flows of this water reach the Earth’s surface through plumes in the ocean, springs and waterfalls on or near the tops of mountains, in geysers, mineral springs, etc. that produce 1000’s of cubic km of mineralised water per year. Consequently, this deep groundwater naturally and continuously accounts for the gradual rise in ocean levels.
Water extraction from deep groundwater bores
Groundwater extracted from the deep, confined fractured rock systems is under considerable pressure and will generally rise in the borehole column to within 50-10m from the surface. In some high-pressure cases, the water will continuously flow out of the top of the bore.
Generally, the bore flow rate will increase from between 30-300% within 6-8 weeks after the bore is commissioned and with regular pumping over this time. There will be minor and some major fissures that have been sealed during the drilling process, and these fissures will open up and water flows from these rock fissures or water bearing clays.
A bore pump system needs to be sized (capacity to lift a volume of water with a given head of pressure) based on the sustainable yield or supply flow rate. This flow rate is usually assessed through a pump test after the bore hole is completed. Also, it can be expected that water quality will improve over time as fresh water from the deep, confined aquifer overwhelms any saline or heavy carbonate inflows from the shallow groundwater system. Similarly, any undesirable shallow groundwater inflows can be sealed off in the commissioning of the bore, as required.
A bore pump can be located at a depth of about 100m into the borehole column provided the top water level is less than 50m from the surface.